The brain is responsible for thinking, combining mental processes such as sensory integration, perception and working memory, grouped together under one broad definition: cognition. The holy grail of neuroscience is to understand how the brain encodes cognition, unifying many specific processes into a coherent intellect. The biggest obstacle in achieving this challenge is the complex nature of the brain, an extremely complicated organ containing billions of neurons entangled together forming a dynamic, ever-changing network. Even more overwhelming is the plastic and dynamic nature of the brain, constantly changing and forming new synapses, knowledge and experience.
There are no two similar brains on earth, and even the same brain can give different outcomes to seemingly identical incoming stimuli. Neuronal populations are multidimensional, merged in an eternal, ever-changing loop between the external and internal worlds.
In the lab, we are interested in studying how the brain encodes
cognition at the brain-wide level. We adopt a mesoscale approach in which we aim to simultaneously image as many brain areas as possible as mice perform complex behavioral tasks involving different cognitive function. Complementing the mesoscale approach, we use multi-area two-photon microscopy, optogenetics and labeling techniques to dissect the relevant neuronal sub-populations responsible for different cognitive functions. Our goal is to obtain a cognitive brain-wide map that will aid in understanding cognition as a whole in both the healthy and diseased brain.
Example study: Locating short-term memory in cortex
An example study is finding the cortical location of short-term memory. To address this question, we trained mice on a memory task and simultaneously imaged the whole dorsal cortex. We found very surprising results in which short-term memory was located at very different locations based on the internal strategy of the mouse (See Figure). Thus, cognitive processing is flexible and dynamic requiring us to use wide-field and flexible approaches in order to obtain a comprehensive understanding.
Figure legend:
A) Mice were trained on a whisker dependent go/no-go task with a short-term memory (STM) component, while imaging the whole dorsal cortex and monitoring body movements.
B) Body movement vector in two example Hit trials, one active and the other passive during the sensation period.
C) STM maps in a single active or passive trial, showing a dissociation of frontal M2 and posterior P respectively.
Our research focuses on different aspects of behavioral training, mesoscale imaging and microscale investigation:
Training mice on different cognitive tasks
One of our main goals is to understand cognition as a whole rather than focusing on a specific aspect of cognition. To do this we aim to train mice on different behavioral tasks, each highlighting a specific cognitive function. Example tasks are go/no discrimination with delayed response, delayed match-to-sample task and a two-alternative force choice task.
Mesoscale imaging techniques to image many brain areas
We use
wide-field imaging to simultaneously image large parts of the cortex. This can be done for either one or two cortical hemispheres. We can image calcium dynamics from up to 31 different cortical areas as mice perform a complex task. In addition, we aim to image subcortical areas using a
multi-fiber approach (Sych et al. 2019). A combination of both cortical wide-field imaging and multi-fiber photometry in one mouse can enable us to maximize our observation across the brain. Using transgenic mice, we can target different neuronal subtypes with a calcium indicator, for example different inhibitory subtypes or different cortical layers. Thus, we are able to compare the brain-wide activity of different neuronal subtypes to unravel the global mechanism of different cognitive functions.
Figure legend: Cortical functional mapping and area definitions
a, Mapping session examples including five stimulus modalities: whisker, visual, auditory, forelimb and hindlimb (Methods). Mean activation maps are shown for each stimulus type for two example mice. Color denotes normalized fluorescence (ΔF/F).
b, Full names and abbreviations of 25 areas. Areas were divided into auditory (green), association (pink), somatosensory and primary visual cortex (blue) and motor (red) cortices.
Microscale investigation to dissect a specific sub-population
Complementing the mesoscale approach, we will use a microscale approach to target specific areas of interests. We use a state-of-the-art
multi-area two-photon microscope to image single cells in multiple non-contiguous areas. We can also label different cells based on their
projection output and thus start segregating neuronal population into different sub-populations. To test causality, we use
optogenetic manipulation to perturb specific neuronal sub-population as mice perform the task.
Neuronal circuits of social interactions in wild-type and schizophrenia mouse model
Social interaction is a key function for our daily life, and aberrant in many disorders such as autism and schizophrenia.
We use a novel method of chronic implant - an optical fiber array, directed to many brain areas simultaneously, that enables us to study the brain network in freely moving mice. We aim to study the brain-wide social and cognitive networks in mouse model of schizophrenia and autism
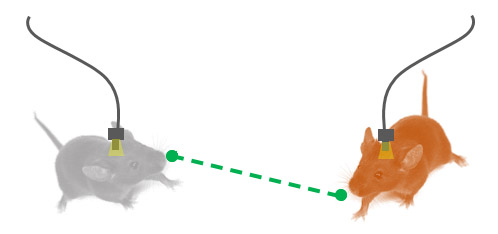